Capabilities
World-class science & cutting-edge instruments
Techniques & Instruments
ECFP has access to a wide range of capabilities for characterising complex fluids. Most of these instruments are situated in the School of Physics and Astronomy, but we also regularly collaborate with other groups across the University to access wider facilities when required.
A full list of equipment can be downloaded at the bottom of the page. If you would like any further information about the capabilities we have or how you can access them, then please contact us.
Capability Highlights
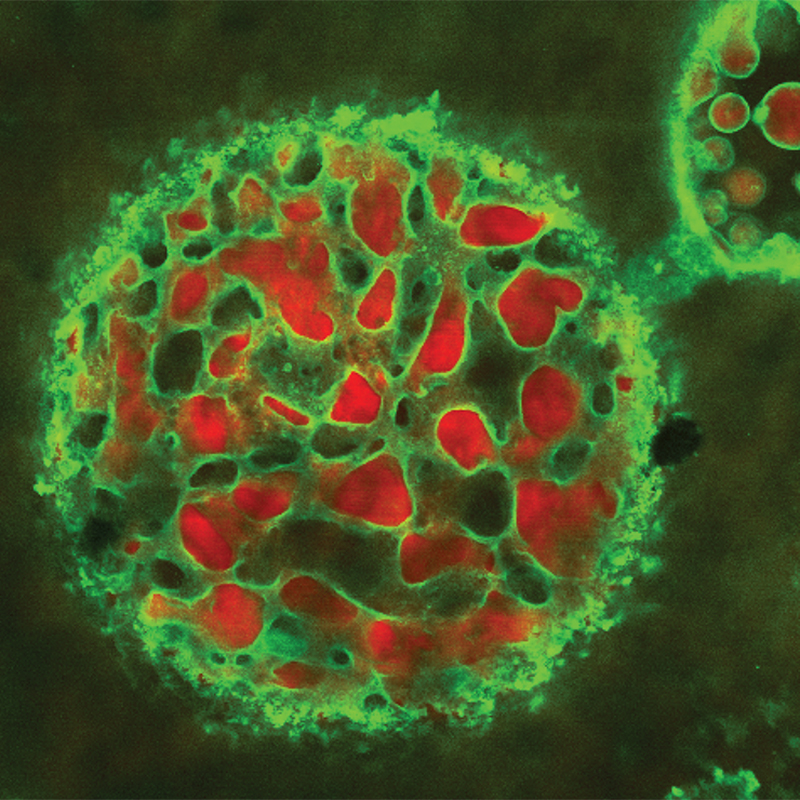
Rheo-confocal microscopy
Physical & Chemical Properties
We have techniques and equipment to characterise the physical properties of individual components so that we may understand how they interact and link this to the architecture of the microstructure observed in a product.
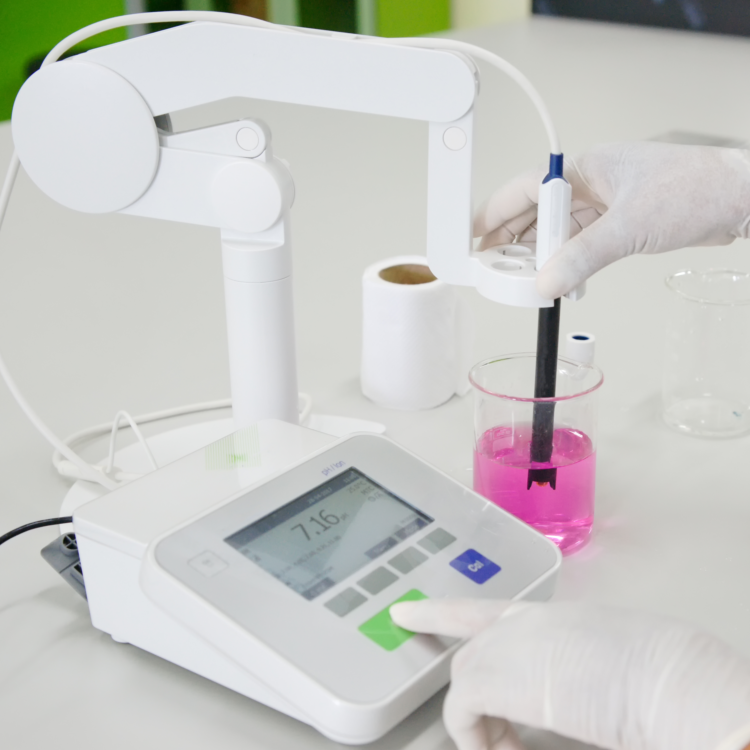
Thermal analysis enables us to probe the thermal behaviour of our system and understand the interactions between different components; how does it respond to heating, cooling or prolonged periods at a certain temperature. In formulation science, these techniques are invaluable to understanding the stability of the product, for example.
A certain product may require a particular microstructure, which may only be stable at certain temperatures, and differential scanning calorimetry (DSC) enables us to understand what temperature range this structure is stable within. Conversely, in food applications, you may want to design the product such that it melts at a certain temperature to provide the desired sensory properties.
Our state-of-the-art TA DSC 2500 enables us to answer all these stability questions and is a great complimentary technique to understanding the structure of the sample. It’s capability of performing modulated DSC opens the door to studying more complex, multicomponent systems, whereby different types of thermal events can be separated.
DSC can be coupled with other instruments at ECFP to maximise outputs. For instance, combining DSC with our Nano Isothermal Calorimeter enables us to understand the interactions between components in our sample. We can then answer questions such as: how does a system respond as we add another component in to the formulation, what mechanisms are at play? Alternatively, combining with rheology highlights how the thermal response of the sample affects its viscosity and rheological properties.
Isothermal Titration Calorimetry (ITC) is a quantitative technique that can be used to study a variety of binding interactions in solution (e.g. protein interactions) to help understand their functions and mechanisms at the molecular level. It provides a complete thermodynamic understanding of a binding reaction. Historically it has been used to study the interactions in biological systems but it is being increasingly applied to the study of binding interactions involving synthetic systems too.
Our optical tensiometer can be used to measure wetting of a substrate through the contact angle of a droplet on the substrate and to measure the surface tension of an interface as a droplet of one liquid is suspended in another. We also have a Spinning Drop Tensiometer to measure very low surface tensions.
Using our Langmuir trough, we can measure the surface pressure of complex liquid interfaces, such as monolayers of proteins, lipids and colloidal particles at a liquid-gas or liquid-liquid interface. It enables precise control of monolayer thickness, homogeneous deposition of the monolayer over large areas and the possibility of making multilayer structures with varying layer composition.
Our Malvern Zetasizer Nano-Z can determine the surface charge of particles and polymers from their mobility as they move through an electric field. Quantifying this charge is important as it can cause flocculation, provide stability or introduce dielectric interactions in a formulation.
We have a Lloyd Instruments/Ametek LS5 universal testing machine, with a variety of compatible apparatus and attachments to facilitate characterisation of both liquid and solids.
Compression and tension tests
We have a range of conventional testing equipment such as compression plates, tension grips and 3-point bend apparatus, in addition to more specialised equipment such as cutting devices replicating cutting of food stuffs on the process line.
Observing stress
Utilizing a polariscope and exploiting the photo-elasticity of various materials, we have a range of materials and geometries that enable stresses to be observed during testing, from deforming a single sphere to observing stresses during extrusion.
Structural Characterisation
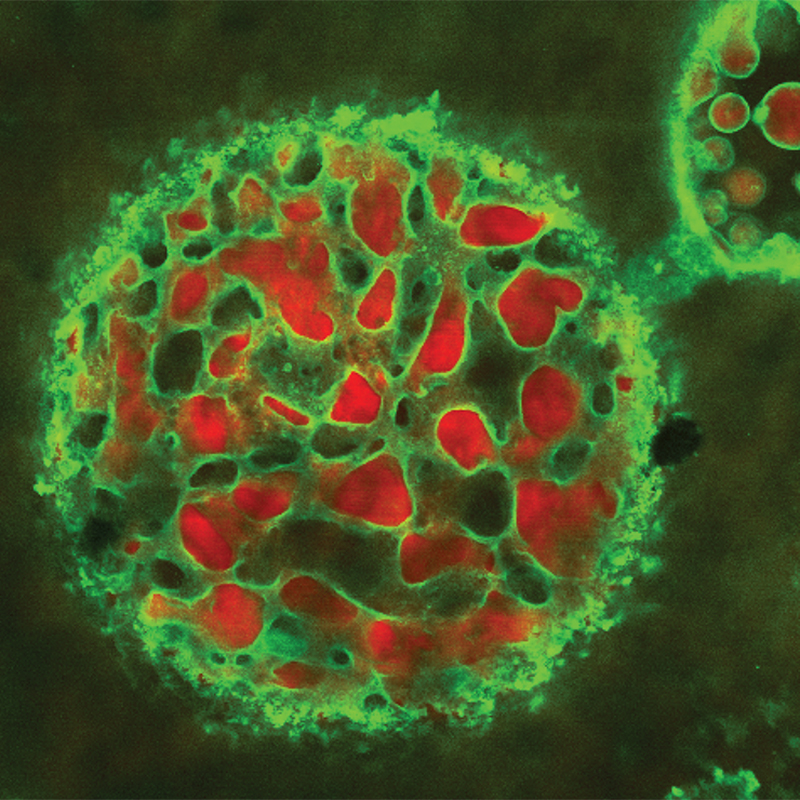
The structural features of components can explain the properties of a product as a whole. By characterising the structure of individual components, we can understand how they interact and link this to the architecture observed in the product’s microstructure. This additional understanding can lead to product improvement and optimisation. For example, characterising the relative sizes of components within a formulation can help determine the root cause of any observed instabilities. The techniques below aid us in characterising individual components, interfaces and microstructures.
Dynamic Light Scattering (DLS), in which we measure the fluctuations in light intensity at a given angle, allows us to probe timescales related to the Brownian motion of particles and obtain a hydrodynamic radius (size range 5 to 500 nm).
Our Mastersizer 3000 laser diffraction particle size analyser allow us access to larger particle sizes through the Fraunhofer approximation of Mie scattering (particle size range 100 nm to 1 mm). We often use this technique to characterize emulsion droplets or aggregates.
Through tagging components (e.g., oil, water, surfactants) with different fluorescent dyes we can identify their location in a product’s microstructure using the multi-wavelength capability of our Zeiss confocal microscope. Seeing this architecture within a product provides a better understanding of its performance and stability.
Our cryo-FIB SEM (Zeiss Crossbeam 550 focused ion beam-scanning electron microscope with cryogenic attachment from Quorum Technologies) provides a three-in-one imaging facility for opaque soft matter.
FIB is a powerful technique that uses a focused beam of charged atoms (ions) to cut and section specimens very accurately inside the SEM. This not only allows users to expose desired sample sections at will, but to also build up a complete three-dimensional picture (literally) by imaging the sample section by section to a resolution of ~1 nm. The microscope also offers elemental contrast and identification, fast and accurate milling and patterning using the focussed ion beam (FIB), nanoscale tomography, TEM sample preparation and the ability to work on liquids, gels, polymers, biological matter and many other materials using the optional cryo mode.
Cryo-SEM-FIB is a relatively new technique: there are only a few current instruments in the UK and the availability of this combined suite of instruments is transforming the ability of soft matter scientists to see inside their samples routinely. Our dedicated cryo-FIB SEM instrument scientist, Fraser Laidlaw, supports scientists wishing to make use of this facility.
More detailed information and example images can be found on our dedicated cryo-FIB-SEM page.
Characterising Dynamics
Characterising dynamics can give key information for the way a product changes when a formulation or process change is underway. Flow behaviour, consumer experience and product stability all depend on the product sensitivity to dynamics. Several techniques are available including Diffusing Wave Spectroscopy and Dynamic Differential Microscopy as well as rheoimaging and rheology.
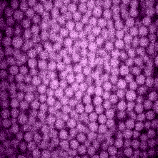
We have access to a suite of rheometers and can apply stress and strain controlled deformation through continuous flow, creep, stress relaxation and oscillatory rheology from small strain through to LAOS mode. We can also undertake interfacial rheology measurements to understand viscoelastic properties at interfaces (e.g., for proteins, lipids, colloids or surfactants at interfaces).
To understand a product or formulation, it is important to link its microstructure and dynamics with its macroscopic properties. Our rheo-imaging facility has a fast confocal microscope (up to 100 fps) coupled to an Anton-Paar rheometer. Simultaneous measurements allows us to measure the rheological response of materials and correlate this with changes in microstructure.
This technique adapts a conventional rotational shear rheometer, usually used to measure how bulk liquids flow, to instead shear oil above a stretched-out water interface, mimicking a droplet. This stresses the interface and confocal microscopy is then used to track tracer particles and measure the strain and structural response. By changing the flow of the oil different stresses can be applied to match a wide range of applications or processing stages in a single technique over a greater range than typical interfacial shear techniques.
Read more about our work developing this technique in a recent publication.
We have various extruders, simulating many industrial extrusion and injection processes. We can control/monitor extrusion rate, pressure and temperature. Due to the range of extrusion equipment we have developed, we can accurately measure extrusion characteristics of a wide range of materials. Current extruder barrels range from diameters of 12 to 50 mm, die diameters range from 0.3 to 18 mm, and die lengths from 3 to 500 mm. We also have complex die geometries including multi-hole dies and 3D printed dies that mimic natural porous materials with interconnecting pores. Filter plates can also be used to examine filter pressing or cake expression. Bespoke imaging set-ups to observe the flow regimes within the extruder or monitor extrudate surface quality in real time are also available.
Differential dynamic microscopy is an imaging technique that characterises the dynamical properties of colloidal suspensions over a wide range of particle concentrations. In particular, DDM can be used to study the behaviour of both swimming microorganisms (e.g., bacteria and microalgae) and artificially propelled microscopic swimmers (e.g., Janus particles). Using low-magnification images from standard microscopy, DDM can quickly determine changes in the motility of large populations these microorganisms in response to stimuli, anatagonists or environmental conditions. ECFP scientists have been extensively involved in the development of this useful technology and have significant expertise in adapting it for use in a variety of different systems and microswimmers.
With Diffusing Wave Spectroscopy, the dynamics of turbid soft matter can be gathered and analysed over time. This technique can be also used to determine the size of emulsion droplets and bubbles to characterize coalescence over a period of time. Changes in diffusivity due to aggregation (e.g., the early signs of gel collapse) can also be identified.
Optical Coherence Tomography (OCT) is an optical version of ultrasound, using light to create 3D images of samples with up to 3μm resolution and a 16 mm x 16mm field of view. It can also be used to image flow e.g., with droplets and evaporating films, and will penetrate a few mm into highly scattering samples. No sample preparation is needed and the technique is also fast, non-contact and non-destructive.

Example uses include:
- Flow/evaporation of blood
- Structure of skin and penetration of creams
- Structure and flow of concrete
- Dense particle suspensions
- Pickering emulsions and bijels
- Active materials
- Biofilms
Biological Capabilities
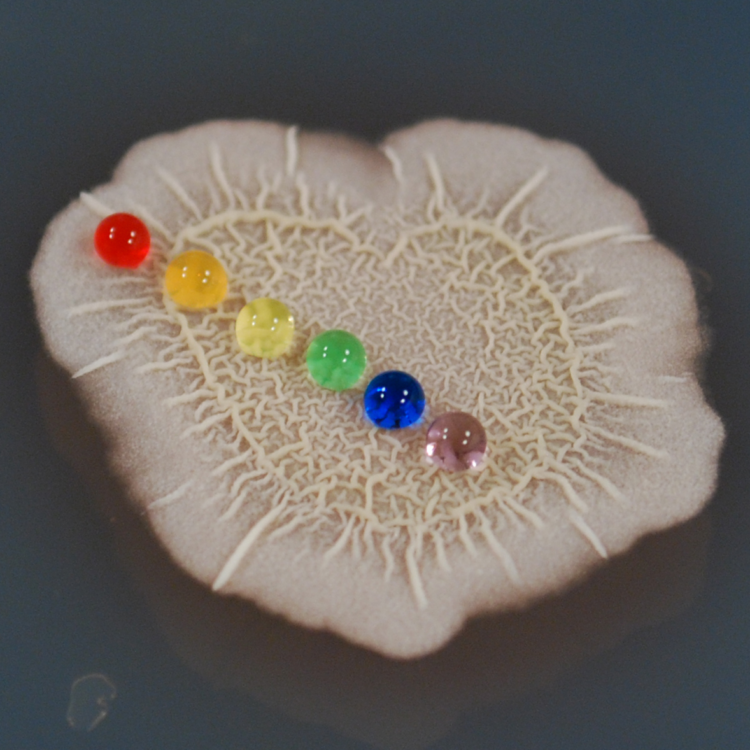
We possess a wide range of biological capabilities and have access to extensive cutting-edge facilities as well as Biosafety Levels 1 and 2 laboratories.
We are open to projects involving laboratory experiments with both category 1 and 2 microorganisms. Our BSL-1 facilities can be used for experiments with model organisms such as Bacillus subtilis and Escherichia coli and our fully equipped BSL-2 facilities can work with bacteria associated with human diseases, such as Pseudomonas aeruginosa and Staphylococcus aureus.
We can run computer simulations of diffusive dynamics, hydrodynamic interactions, anisotropic systems, rare events, biofilm growth, and bacterial population growth in competitive and challenging environments.
For culturing bacteria and growth characterisation we have several plate readers (for measuring optical density, fluorescence, luminescence) and a liquid handling robot for high throughput growth curves. We can grow bacteria in complex media, do fluorescence microscopy, fast confocal microscopy, 3D microscopy, selective plane illumination microscopy (SPIM), scanning electron microscopy (SEM) and cryo-SEM-FIB microscopy. We can also grow biofilms with drip-flow reactors, adapted reactors and microfluidic systems.
We can procure and grow microbiological strains while employing good microbiological practise. We have expertise in optimization of DNA extraction methods, DNA sequencing, sequence analysis and other modern molecular biology techniques. Molecular biology experience is used to construct and modify strains, for example, making deletion derivatives and modifying a strain to enable site specific labelling with fluorophores. We can produce and genetically modify a range of bacteria as well as DNA-based materials with potential pharma and biomedical applications.
We can study bacteria in different conditions. For example, at high pressure using microscope pressure cells, in anaerobic conditions using an anaerobic chamber, in confinement by growing bacteria in microfluidic devices, or inside droplets or in channels made with agarose gel.
Simulation & Modelling
We have a strong community of computer simulation researchers working alongside experimentalists. Numerical simulation provides a tool for studying interactions and dynamics at a level of detail usually inaccessible to experiment. We can ‘switch off’ certain physics in order to isolate a specific mechanism of interest, then systematically add complexity layer-by-layer to build up a working model of the physical system. This approach is complementary to experimental study; indeed the two usually naturally run in parallel with findings from one being used to refine the other, ultimately leading to a comprehensive understanding of the real system.
Dependent on the physical problem being addressed, we select an appropriate level of simulation from a range of techniques in which we have expertise:
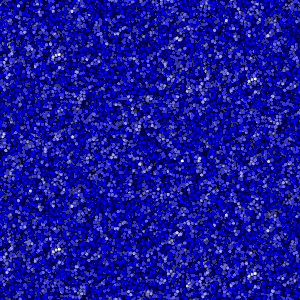
MD simulations – where Newton’s equations of motion are used to propagate a system in time, resulting in a ‘molecular movie’ – are a popular and powerful tool to obtain an understanding of the molecular interactions that ultimately determine the behaviour of formulations. Our researchers have applied MD simulations to study diverse and complex (bio)molecular systems, including protein fibres, protein-DNA interaction and mixed lipid bilayers.
Brownian dynamics is a variant of MD that simulates the trajectories of particles subject to the overdamped Langevin equation. This is particularly suitable for colloidal particles (~10-1000 nanometers) in viscous solvents: we don’t model the solvent explicitly and instead focus on the trajectories of the particles. This allows larger scale simulation of phenomena such as colloidal gelation.
The discrete element method (DEM) is a further variant of MD, this time suitable for granular particles (>1micron). These typically neglect thermal motion and instead focus on contact forces between larger particles: lubrication, adhesion and friction. This allows simulation of bulk rheological phenomena including dense suspension rheology, yield stresses, extrusion and milling.
Chemical & Technical Support
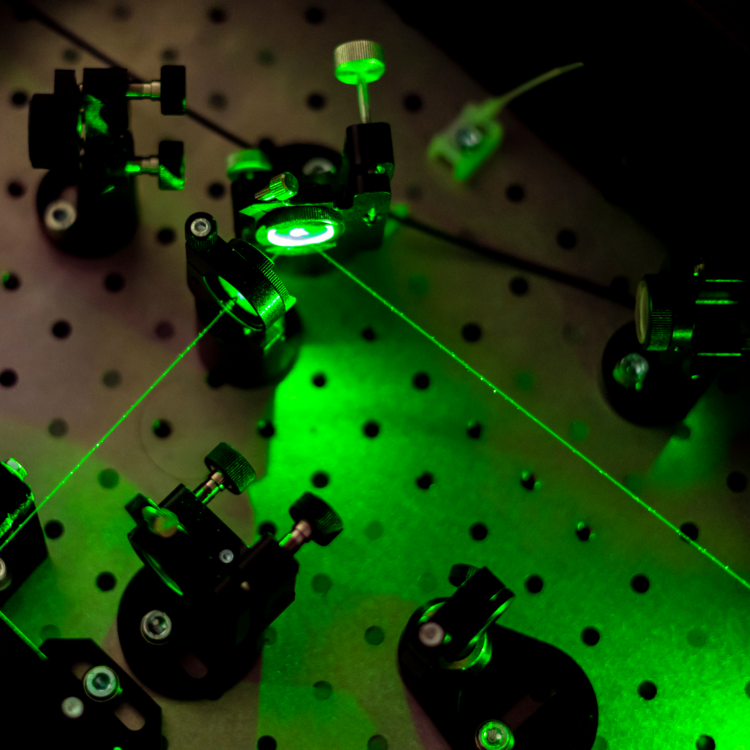
Our scientists are supported by high-calibre experts in optical and mechanical engineering thus enabling us to push forward with adventurous research.
ECFP has an in-house chemist who produces model colloid systems with hard-sphere interactions for the international soft matter community. We often employ these to build experimental models of industrial formulations to develop an understanding of the contribution of other additives on inter-particle interactions and product performance.
We make model PMMA (poly (methyl methacrylate)) particles with hard sphere interactions (sterically stabilised) in organic solvents. Particles can be fluorescently dyed if required and radii range from 40 nanometres to 2 microns. Aqueous PMMA particles are also available and these can be either charge or sterically stabilized. Again these can be dyed if required.
Costs:
Hard-sphere particles in decalin: 10ml of 30%w/w solid £150, 150ml of 30%w/w solid £1500.
In water: 10ml of 30%w/w solid £125, 150ml of 30%w/w solid £1250
Additionally, we can modify the chemistry of surfaces and have the expertise to make a range of novel particles such as magnetic particles, rod-shaped particles, core-shell particles, Janus particles and even motile colloids. Please get in touch if you would like a more unusual type of particle.
ECFP has significant expertise in optical and mechanical design and can support the building of new experiments and the development of prototype instruments.
Through the university, we have access to a Mechanical Workshop. The workshop can design and build services using different materials for a wide range of purposes and produce bespoke equipment to support our research.
Wider Facilities
ECFP can access facilities housed across the University of Edinburgh. Many state-of-the-art instruments and capabilities are hosted by Schools in the Kings Buildings campus. Some of the key facilities within the Schools of Chemistry, Engineering, Geosciences and Biological Sciences are linked below.
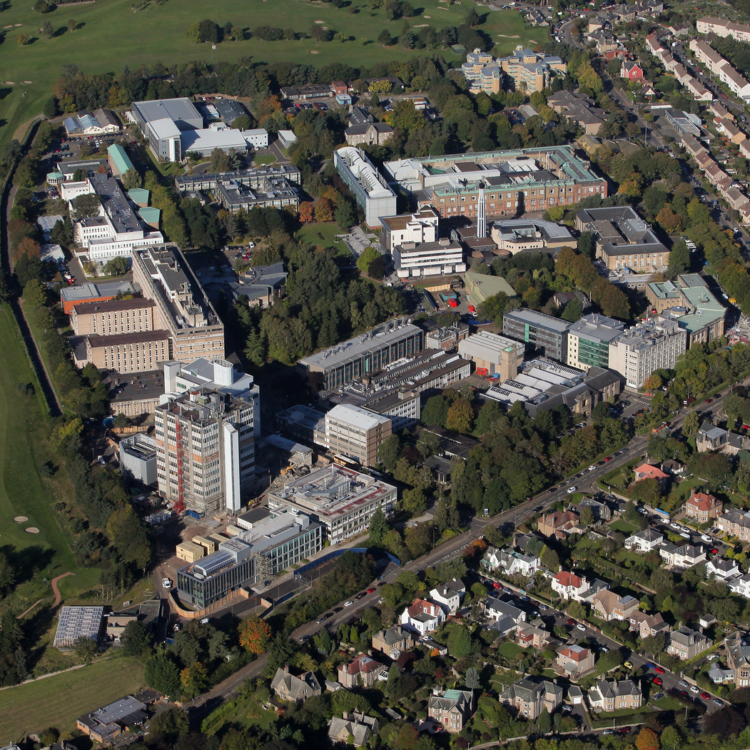
Capabilities List
A full list of equipment and capabilities can be downloaded here. If you require any additional information on these instruments or would like to discuss how ECFP can help better understand your product or process, then please contact us.